In this episode, we peer into plant cells. Researchers are using measurements from single cells to understand which genes help plants grow, get nutrients, weather drought, and more. And eventually, their findings could help us grow better crops, with less impact on our planet. Find show notes here.
Episode Transcript:
Menaka: Above ground, it’s so easy to feel like you know a plant. The leaves! Shoots! The flowers! Certainly, for decades, this is how farmers and plant biologists have gotten to know plants.
But there’s so much more to every plant out there. Below ground, there are roots, of course! And there’s even more to know about plants on a deeper level. How does a plant stay hydrated? Or pull in nutrients? Or avoid disease?
All of these things first start at a really small scale. In this episode, we’re opening the gate to the realm of the very, very small. And we’re headed to the doorstep of a plant cell.
Because that’s where you have to go to really, really know a plant. Behind the tough, cellulose doors of their cell walls.
And with new ways of studying plants at a single cell level, researchers are learning more about all kinds of plants.
So let’s head in!
<<music>>
Menaka: This is Genome Insider! Where we tag along with JGI-supported researchers who are studying all kinds of things, and see how their work could advance clean energy and protect our environment. I’m your host, Menaka Wilhelm.
Today, we’re peering into plant cells. At this tiny, cellular level, there’s a lot to learn. As our crops grow into food and fuel, there is so much happening inside each of their cells.
And we’ll take a look at a few different ways researchers are working to understand big-scale questions by starting at this tiny scale. Because, to grow better crops, there are lots of different places to start.
Here’s one. Like all living things, plants need nutrients to survive. Farm soils are often low in nutrients. So we use fertilizers, like the chemicals nitrogen and phosphate, to grow crops. But pretty much every step of the way, these fertilizers are hard on the environment. Phosphate fertilizer comes from actual rocks, and we make most nitrogen fertilizer using natural gas. To transform raw materials into fertilizers, we burn fossil fuels, and eventually, we’ll run out of all of these ingredients.
Then, commercial fertilizers boost soil nutrients, but they also release more greenhouse gases as they break down in soil. And fertilizer rarely stays in its field. It ends up in our waterways, and affects ecosystems far beyond farms.
Margot Bezrutczyk: We are really in trouble when it comes to fertilizer use.
Menaka: Margot Bezrutczyk is a plant biologist at the JGI. But fertilizer is only one way to deliver nutrients to plants. In healthy soil, microbes like bacteria and fungi help ferry nutrients and water to plants. If we could reliably boost the way those microbes interact with plants, plants could rely on microbes, rather than fertilizer, for some of their nutrients. So there’s certainly a large-scale problem here, but there are also still options to explore. Some of them take us back to our very small, cellular scale.
Margot Bezrutczyk: And I hope that we can engineer plant microbes to be part of the solution.
Menaka: We’ll get back to the plant microbes Margot is working on soon – but first, let’s take a look at the way she’s studying these plant-microbes. She’s zooming way in, using techniques called single-cell genomics. These kinds of experiments are opening doors for researchers to understand more about plants than ever before.
<<music>>
Menaka: When you’re trying to get a snapshot of what a plant is up to, the way you measure samples affects the picture that you get. And studying plants at a single cell level, like Margot is doing – it’s new. She and other Berkeley Lab researchers are fine-tuning these techniques. With more information at this tiny scale, they’re hoping to grow better crops, with less environmental impact. Here’s Ben Cole, who is also a plant biologist at the JGI.
Ben Cole: Traditionally, what you would have to do is, take a root, and, kind of grind up all of its cells together. And, then, take that collection of tissue, and profile that as a single sample.
Menaka: In that case, all of the different cells in your sample get smeared together. So the picture you get from this kind of measurement is maybe something like an impressionist painting. I think of one of Monet’s paintings. It’s Waterloo Bridge at Sunset. You can find a link to it in our show notes. When you look at that painting, you’re certainly seeing something – a bridge, with light glinting off of it, against the water and sky. It’s beautiful. But it’s hard to make out much more detail than that. The brushstrokes are broad, and it’s a little bit blurry.
Ben Cole: But recently, you know, there’s this explosion of technologies that allow us to look at each individual cell, one at a time.
Menaka: These single cell genomics techniques give you individual readings from many different plant cells. And that gets you a picture that’s much more like a pointillist painting. Like Georges Seurat’s classic, A Sunday on La Grande Jatte, from 1884. Also linked in our show notes. In that painting, you see a bunch of different things happening in a park. Rowers in their boat on the pond, kids skipping through trees, ladies with parasols, even a monkey on a leash. And it’s hard to tell at first, but every single piece of the painting is made up of tiny, individual dots. There’s less haze, and much more detail to zoom in on.
So single cell genomics techniques are giving researchers this kind of pointillist view of plant processes. With this kind of image, they’re getting loads more detail than before. And they’re tackling all kinds of questions. Ben recently organized a symposium about these techniques at The American Society of Plant Biologists annual meeting, and I had a chance to hear from the presenters about where single cell genomics fits into each of their work. Here are two researchers from that symposium who happen to work on the same plant: corn. We talked outdoors at the conference, so you’ll hear a bit of outdoor noise.
<<music>>
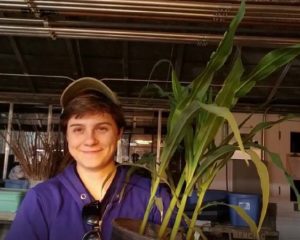
Diana Ruggiero of Oregon State University with a few of the corn plants used to understand leaf vasculature.
Diana Ruggiero: I’m Diana Ruggiero. I’m a PhD student at Oregon State University in the Leiboff lab. I study Zea mays, which is also known as maize, also known as corn. In particular, we’re interested in the veins in the leaves of the plant. There’s a couple of questions we want to answer. One of them being, which cells actually become veins. So that’s something single cell sequencing is really well situated to interrogate, is what has to happen genetically for one cell to turn into another cell type, and where that signal comes from.
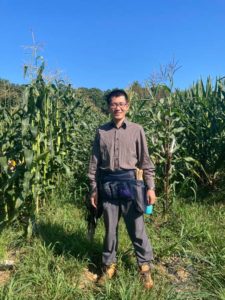
Xiaosa Jack Xu of Cold Spring Harbor Laboratory uses single cell genomics to understand cell development in corn (Maxwell Fehrs).
Xiaosa Xu: I’m Xiaosa Xu. You can call me Jack, I’m a postdoc from David Jackson’s lab, at Cold Spring Harbor. We focus on a crop plant called corn, which is, if you eat sweet corn, that’s our major working organism. Generally I study development in plants, and then specifically I work on the plant stem cells. So that’s very important for the plant architecture and also important for crop productivity. So the single cell RNAseq or genomics is really powerful. And now I can check many different cell types in just one experiment. And then based on the gene expression profile, we can really see all distinct cell types and then identify those cell types’ specific genes.
Menaka: At the single-cell level, it’s possible to get that pointillist-level picture of why specific plant cells change and grow the way they do. So to get back to Margot Bezrutczyk, she’s looking at how plant cells change when they interact with helpful microbes, to try to find ways of delivering nutrients to plants, with less fertilizer. And she’s looking at a really specific plant-microbe relationship, between one plant and one fungus. The plant is related to alfalfa. It’s called Medicago truncatula. And with a name like that, it could certainly be a spell in Harry Potter, if it ever gets tired of being a real plant.
Margot Bezrutczyk: It kind of looks like clover. It has these trifoliate leaves and very small yellow flowers.
Menaka: She’s looking at how that plant interacts with one microbe in the soil. It’s called Arbuscular mycorrhizal fungi. Let’s start with why we call this microbe arbuscular. When it grows alongside a plant, it actually grows right into a plant’s root cells. And the bit that grows into the plant? That is called an arbuscule.
Margot Bezrutczyk: It’s like a tree shaped structure inside of the cells.
Menaka: And that arbuscule is like a transfer station, between the fungus and the plant. That’s how it delivers nutrients to the plant, through its roots. This fungus also has mycorrhizae – that’s what makes it mycorrhizal. And mycorrhizae is a network of small filaments. They form almost a net around each tendril of a plant’s roots.
Margot Bezrutczyk: It’s very, very fine filaments that you cannot see by eye.
Menaka: So between being arbuscular, and mycorrhizal, these fungi are very helpful to plants.
Margot Bezrutczyk: They provide plants with phosphorus. They also provide plants with some nitrogen, and recently it’s been demonstrated that they provide plants with water in very dry soils, partly because they act as an extension of the plant’s roots system.
Menaka: So plants with arbuscular mycorrhizal fungi have a big leg up. Besides getting a better supply of nutrients and water, they’re better at resisting disease, also. To really understand this, Margot is looking at how different cell types in a plant’s roots react to this arbuscular mycorrhizal fungi. Because she’s using single-cell techniques, she’s getting measurements from all kinds of different root cells, all at the same time.
Margot Bezrutczyk: And so for the first time, we’re able to look in an unbiased manner at all of the different cell types and how they react with this.
Menaka: So there’s that pointillist view, rather than an impressionist painting, of what’s going on. And there’s even more benefit to these single-cell approaches. Without single-cell techniques, Margot would have to decide what to measure, ahead of time. Which narrows the possibilities for discovery. Treating each cell individually – as its own dot – leaves more room for surprising results. And the way these measurements work is pretty fascinating – we’ll get to that in just a bit.
<<music>>
Menaka: First, a little about the JGI. The JGI is a taxpayer-supported user facility. So supporting all kinds of scientists’ work is what we do. We have projects in genomic sequencing, synthesis, transcriptomics, metabolomics, and natural products, in all sorts of non-human organisms.
To work with us, scientists can submit proposals to the JGI Community Science Program.
Right now, we’re looking for projects in Functional Genomics. Maybe you’re looking at genes and pathway synthesis, strain engineering, metabolomics, or plant-microbe interactions? Submit a proposal – they’re reviewed twice a year!
You can find more information about each of these at our website. There’s a link in the show notes.
<<music>>
Menaka: OK, so back to single-cell genomics. As Margot tackles that fertilizer problem, she’s looking at plant roots – and so are a bunch of other scientists. So here, we’ll check back to a few of the presentations at the American Society of Plant Biologists recent single-cell symposium.
<<music>>
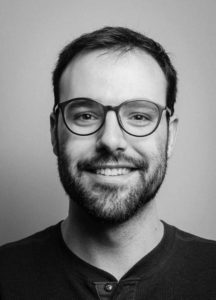
Alex Canto Pastor of UC Davis, who uses single cell genomics in his experiments in tomato plants. (Bruno Santos).
Alex Canto Pastor: My name is Alex Canto. I’m a postdoc at UC Davis. I work at the lab of Siobhan Brady. At our lab, we study plant root development. I specifically work in tomato and we focus on a cell type that is called the exodermis and it deposits apoplastic barriers that help protect the root against the external environment. Our overarching goal is to try and understand these unexplored cell types and the best tools are always gonna be the most agnostic ones, with single cell as a prime example of that.
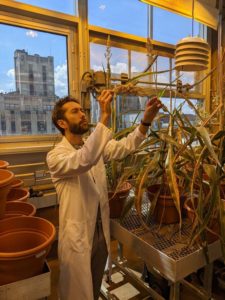
Bruno Guillotin of New York University, who studies crops like sorghum, pictured, with single-cell techniques (Laura Lee).
Bruno Guillotin: My name is Bruno Guillotin. I’m a postdoc in the lab of Kenneth Birnbaum at New York University. I’ve been working on single cell and single nuclei RNAseq in multiple crop species, such as corn, or like sorghum or Setaria, which is kind of a type of millet. We try to use single cell to identify the different cell types present in the roots of these plants and try to compare why, maybe, sorghum is more drought resistant or why maize is so good at harvesting nutrients from the soil.
Menaka: So Margot’s work fits into this bigger picture of improving crops, starting at this teeny tiny level. Let’s get back to her research on that plant-fungus relationship. Our fungus is that arbuscular mycorrhizal fungus. It grows in and around a plant’s roots, and shares water and nutrients. And if we could help more plants get into these nice plant-microbe relationships, we could fertilize them less.
So Margot is looking at how this plant-fungus interaction starts. To see what she’s actually measuring, we have to go a few steps back.
For any plant to do pretty much anything, it activates genes, sections of its DNA. Then, it transcribes those genes into messenger RNA, or mRNA. And from mRNA, a plant makes proteins, which snap into action. Proteins send signals, get reactions going, they generally make things happen.
But that protein action part would never happen without mRNA. So taking a look at a plant’s mRNA is a good way to know what it’s up to. And that’s what single cell genomics ultimately measures – mRNA, from individual cells.
There are two different techniques Margot uses in this work. They both measure mRNA, but with different goals, and in each technique, special barcodes keep track of individual cells.
The first technique is called single nucleus sequencing, or single cell sequencing, because you measure genes from cells, or from one compartment of a cell, the nucleus. So you take a plant sample and break it up a bit, so you get kind of a soup of all the plant cells. But each individual cell stays separate and intact.
Margot Bezrutczyk: And then you run them through this microfluidic device,
Menaka: And that microfluidic device does something that is both amazing and still tiny. It gives each cell from your sample a barcode. The barcode is made of DNA. So you sequence the genetic material – the mRNA from all these cells from your sample,
Margot Bezrutczyk: And then what you end up with is, a library of mRNAs where each mRNA has a barcode, that you can trace back to the cell that it came from.
Menaka: So it’s possible to see exactly which kinds of cells are activating certain genes. Which is pretty amazing.
Margot Bezrutczyk: But one thing that we can’t tell when we’re looking at this, is that we don’t know where any of this is happening.
Menaka: And that brings us to the second single cell genomics technique Margot is using. This one is called spatial genomics. As any realtor will tell you, location is key. This is especially true for that plant-fungal relationship that Margot studies – how arbuscular mycorrhizal fungus moves into root cells. Because while the fungus might grow into one kind of cell, that cell’s neighbors probably helped out, too – even if they’re not hosting any fungus.
Margot Bezrutczyk: And so we need to know, what other cells might be involved that are directly adjacent to where this interaction is taking place.
Menaka: To understand that, Margot makes kind of a map of a root sample, before sequencing its cells mRNA. She cuts the root into extremely thin slices.
Margot Bezrutczyk: So they’re, they’re between like 10 and 16 microns thick, so very, very thin. And then we take that very, very thin frozen section, and we lay it on these slides
Menaka: And these slides have a special grid, where each square has a barcode.
Margot Bezrutczyk: So just like with the single cell sequencing, once we create the library, we have mRNAs that we know where on the slide they came from, based on that barcode.
Menaka: And right now, the resolution of this grid is about 50 microns by 50 microns. That’s a little smaller than the width of a human hair. The goal is to eventually be able to see with even smaller resolution – to make a pointillist picture with even smaller dots! Because there are still so many pieces to understand about this plant-fungus relationship.
Margot Bezrutczyk: All of the receptors involved in sensing this interaction, the way that the cell wall is altered and modified to support the continuation of this interaction, the feedback loops that are preventing the interaction from taking place. Without those pieces of information, you can’t engineer this to be more efficient or more widespread.
Menaka: But already, studying these plant-microbe relationships at a single-cell level has been interesting.
Margot Bezrutczyk: There’s been some surprises in the data. You know, there have been some genes that we already knew, based on previous studies, were up-regulated during this interaction.
Menaka: And because they had a sense of those genes that were active, people had ideas about which cells would be turning on those genes.
Margot Bezrutczyk: And it turns out there’s actually a lot of other cell types that seem to, also over-express that gene under these conditions.
Menaka: And for Margot, Ben, and the other scientists we heard from, this was the part of single cell genomics that they were most excited about. That keeping cells separate, and measuring them all at once, allowed them to ask much more open-ended, exploratory questions, and get surprising answers.
Margot Bezrutczyk: It seems like there are things that we didn’t know to look for before that are now revealing themselves to us based on this unbiased approach.
Menaka: And with so many questions about how these plant-cell-level processes could improve giant, crop-scale level issues – certainly, the more information, the better.
<<music>>
Menaka: That wraps up our peek into plant cells! We’ll be back in a month with a look at a fungus that manages to exist in a very hostile place: Antarctica.
This episode was written and produced by me – Menaka Wilhelm, with production help from Massie Ballon, Allison Joy, and Ashleigh Papp.
Many thanks to Sarah Black with the American Society of Plant Biologists, and to our researchers: Ben Cole, Margot Bezrutczyk, Xiaosa Xu, Alex Canto Pastor, Diana Ruggiero, and Bruno Guillotin.
If you liked this episode, help someone else find it! Tell them about it, send a link over, or leave us a review wherever you’re listening to the show.
Genome Insider is a production of the Joint Genome Institute, a user facility of the US Department of Energy Office of Science located at Lawrence Berkeley National Lab in Berkeley, California.
Thanks for tuning in – until next time!
Show Notes
- Monet’s Waterloo Bridge at Sunset (1904)
- Seurat’s A Sunday on La Grande Jatte (1884)
- Submit a proposal to work with the JGI
- Margot’s 2021 Berkeley Lab SLAM talk
- The JGI’s Genomics of Plant-Microbial Interactions group
- Plant Single-cell Solutions for Energy and the Environment (Workshop report)
- JGI Blog: A Plant Root Atlas for Tracking Developmental Trajectories
- Ben Cole’s DOE Early Career Award
- Our contact info:
- Twitter: @JGI
- Email: jgi-comms at lbl dot gov
Genome Insider is a production of the Joint Genome Institute.
This episode uses two pieces of music from Free Music Archive: