Researchers looked across many organisms to see that aminotransferase enzymes have evolved mix-and-match characteristics — creating many different ways of processing nitrogen.
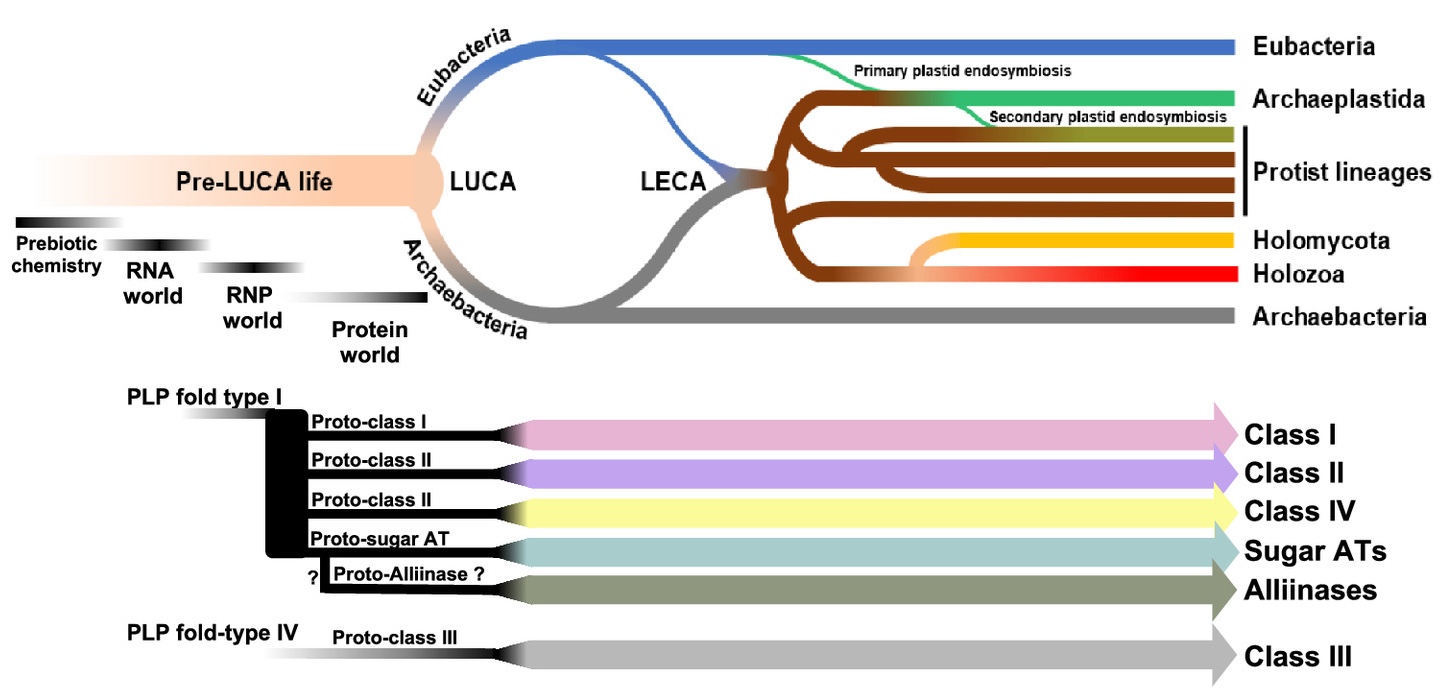
A look at how aminotransferases (ATs) have evolved from the origin of life to today. Pre-LUCA life likely had nonenzymatic, RNA, or RNP-based transamination. At the interface of RNP and protein worlds, protein-based transaminases appeared in the form of proto-ATs classes. Proto-AT classes were inherited by LUCA and its descendants. (Kaan Koper and Hiroshi Maeda)
To boost crops more efficiently in the future, the evolutionary past may hold key insights. The way that plants process nutrients has a rich back story — they rely on enzymes that have been evolving for billions of years. However, these enzymes are often loosely understood, leaving potential targets for crop engineering untouched.
Research recently published in The Proceedings of the National Academy of Sciences bridges this gap, by tracing the evolution of a specific enzyme family called aminotransferases (ATs). These enzymes play a crucial role in how an organism processes both carbon and nitrogen.
This research team combined computational and experimental approaches to look at how this enzyme family evolved across 90 species. “One unique thing about this work was our focus on all the kingdoms, including animals, plants, bacteria, archaea and fungi. We thought this kind of analysis would provide a framework for the evolution of this enzyme family,” said Sang-Woo Han, one of the co-first authors of this work.
Han is an Assistant Professor at Konkuk University in South Korea as well as a faculty affiliate in the Environmental Genomics and Systems Biology Division of Lawrence Berkeley National Laboratory (Berkeley Lab). He completed this work with collaborators at the University of Wisconsin-Madison as well as the U.S. Department of Energy (DOE) Joint Genome Institute (JGI), a DOE Office of Science User Facility located at Berkeley Lab.
In addition to providing metagenome mining for computational analysis of the AT enzyme family, the JGI provided DNA synthesis capabilities to enable functional testing of these enzymes.
Yasuo Yoshikuni, head of the JGI’s DNA Synthesis Science program, underscored the value of taking a look at the AT family. “Essentially, the aminotransferases that we studied are involved in transfer of the amine group, or the N group, to a variety of different molecules. So they’re involved anytime you make an amine-based molecule — a very critical role,” he said. These enzymes are crucial for creating nucleic acids and proteins, as well as recycling nitrogen.
Now, with insight into a specific enzyme class that’s important to the way many organisms function, they have a sense of why nitrogen metabolisms can be so varied.
As evolution has progressed, organisms have developed metabolisms that function with a toolkit of enzymes. It’s useful to differentiate these enzyme tools into two groups: core metabolism and specialized metabolism.
For enzymes involved in core metabolism, organisms tend to have enzymes evolved to high performance, with specificity — a bit like including hex keys in many different sizes in a toolkit. This makes it possible to process more molecules efficiently, with just the right tool for the job. For enzymes in specialized metabolism, organisms tend to evolve multiple copies of enzymes, similar to including half a dozen slightly different screwdrivers in a toolkit. This way, these important tools are always around, regardless of malfunction or misplacement.
In studying this family of ATs, researchers found that these enzymes have evolved distinctly from both core and specialized enzymes. Even during specializing or multiplying, ATs have maintained functional versatility with fewer enzyme copies. In this way, this family is more like including a Swiss Army knife within a toolkit – a tool with some redundancy, that is both functionally versatile as well as compact. Because these ATs can facilitate reactions with multiple types of molecules, they’re versatile in ways other enzymes are not.
“It turns out that all of life created the minimum set of ATs that act together to create the robust network to supply amino acids fast enough for life to grow,” Yoshikuni said.
To gain this insight into the evolution of ATs, this team traced the evolution of these enzymes across 90 species. They queried the genomes of 15 species from each of six kingdoms: eubacteria, archaea, protist, plant, fungi and animal. This analysis led them to identify 2,938 putative AT genes.
Within the AT genes identified across different species, each organism has a relatively small set of around 20 ATs. These ATs have maintained a small and relatively constant number across different kingdoms, with archaea having around 15, eubacteria around 25, and eukaryotes having slightly larger numbers due to their expanded metabolisms. These ATs can operate in a relatively small set because of their unique functional redundancy.
To understand how founder AT enzymes became integral to core nitrogen metabolism, this team built a phylogenetic tree using bioinformatic software called FastTree and identified 62 distinct AT groups. However, no single AT group was conserved across all species — a surprise for an enzyme that plays a central role in metabolism.
This lack of conservation is likely due to the fact that AT enzymes have undergone non-orthologous gene displacements, where they replaced other ATs in different species, seemingly driven by the functional versatility of ATs. This replacement has allowed AT enzymes to evolve in complex ways.
Finally, to take a closer look at the functional redundancy of ATs, this team leveraged the JGI’s DNA synthesis capabilities to express, purify and characterize recombinant enzymes to determine substrate specificity. They found that even enzymes that evolved separately for billions of years have some common signatures of muli-substrate specificity. However, different organisms use different active residues to achieve this, a bit like having slightly different Swiss Army knives — explaining a bit more about why and how nitrogen metabolism is so robust and varied.
Publication
Koper K, Han SW et al. Multisubstrate specificity shaped the complex evolution of the aminotransferase family across the tree of life. Proc Natl Acad Sci U S A. 2024 Jun 25;121(26):e2405524121. doi: 10.1073/pnas.2405524121.