In our warming world, we’ll need corn, sorghum and other crops to grow well in worse conditions: with more heat, less water and less fertilizer. Grasses do better in these conditions, so plant biologists James Schnable, Guangchao Sun and Vladimir Torrres (University of Nebraska at Lincoln) have looked into traits that could transfer from grasses into other crops.
One grass they studied just happened to be the same species that covered World Cup pitches in 2022. Find show notes here.
Episode Transcript:
Menaka: Ahead of the 2022 World Cup, while the rest of the world wondered which countries would qualify, there was a different competition going on, in the turfgrass world: which grass would cover World Cup stadium pitches. Three different grass varieties were in the running: bermuda, zoysia, and seashore paspalum.
The winning grass would beat out its competitors by growing well in heat, and tolerating shade. And better yet, it would grow quickly – with less fertilizer, and less water.
Basically – qualities we might want in any crop that grows in our warming world.
As all three grass varieties went head to head at multiple test sites, experts tallied their turf-ly talents, like density, durability, and growth.
In the end, one grass prevailed. The game and practice fields in Qatar were covered in the same turf. It was a patented variety of a grass called seashore paspalum.
Meanwhile, totally separate from the World Cup – a team at the University of Nebraska at Lincoln had also been thinking about seashore paspalum.
Guangchao Sun: The scientific name is Paspalum vaginatum,
Menaka: That’s Guangchao Sun. He studied this grass for a few years, when he was a postdoc in James Schnable’s lab. Their team was excited about how tough paspalum was. They were trying to understand why.
And in the summer of 2022, they had results ready to publish — right when the World Cup began.
So that’s when these paspalum storylines collided. James Schnable realized paspalum would be on World Cup fields.
James Schnable: The paper was just starting to come out, and oh! There’s this other, completely different story about why this is an exciting plant to be working on.
Guangchao Sun: I was watching a game, and then I got a message from James saying, “Hey, paspalum is actually the turf grass of the World Cup.” I almost jumped, you know,
Menaka: It’s pretty exciting to find out you know the plant covering the field –
Guangchao Sun: Since then, I cannot help myself, but looking at the grass every time when I watch the game. You know, I was a turf watcher, but not the game watcher.
Menaka: And so naturally, you’d tell your friends,
Guangchao Sun: Hey, if you watch the World Cup, pay attention to the turf!
Menaka: Which is the kind of thing that tends to spread!
James Schnable: And so I would get text messages, even when I wasn’t watching games of, “Hey, just saw this game, man, the turf looks great,” and I take no credit at all for the turf, but it was fun that yeah, that was what people were thinking of.
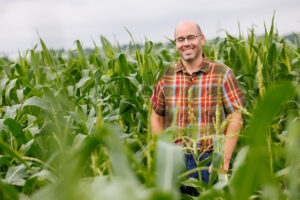
James Schnable, professor of Agronomy at the University of Nebraska at Lincoln. (Craig Chandler, University of Nebraska at Lincoln.)
Menaka: So in this episode – we’ll hear what James, Guangchao and their team found — when they took a look at the species of grass that Argentina beat France on.
Menaka: This is Genome Insider from the US Department of Energy Joint Genome Institute. Where researchers discover the expertise encoded in our environment — in the genomes of plants, fungi, bacteria, archaea, and environmental viruses — to power a more sustainable future. I’m Menaka Wilhelm.
And first, a little groundwork. There’s a big reason that our plant biologists have turned their attention to turf grass. Like they said, they’re not really in the sportsfield scene. They’re really interested in crops. Corn, millet, rice, sorghum — plants that become food, as well as other products, like biofuels.
But they also study grass, because unlike farmed crops, wild grasses can often exist with less water, or more heat, or less nutrients. And that could turn out to be useful for lots of other plants. Because the grass family is a broader group than most people might expect.
Rice, sugar and corn all fall into that large family. So we get food from grass fields, technically. Corn and sorghum also become ethanol biofuel. As time goes on, we’ll need more ways to make sure these grasses can still grow well, in a warming world. Which might shift how you feel about watching grass grow.
And actually — the grass we’re talking about today — seashore paspalum — it grows so aggressively that it must be watched.
Guangchao Sun: I can tell you a story about it.
Menaka: That’s Guangchao Sun again. So several years ago, he had just started as a new postdoc at the University of Nebraska, Lincoln, in James Schnable’s lab.
Guangchao Sun: So I was given a tour,
Menaka: They went all around the greenhouse space, to show off what the lab was working on. Seashore paspalum got a special shout out.
Guangchao Sun: And I was particularly told that, you know, you need to pay more attention to this grass, because this grass grows very fast given the same amount of greenhouse water. And you need to trim it regularly, otherwise the plant will try to invade the pots of adjacent plants.
Menaka: Paspalum can grow outward from its stalk, send out rhizomes, and crawl its way into other specimens’ pots.
Guangchao Sun: And, the greenhouse manager will be mad at you <laughs>. So, just to give you a sense of, you know, this grass grows super fast.
Menaka: And at first, actually, Guangchao kind of figured that growing paspalum wouldn’t really be his problem.
Guangchao Sun: I was hired for another project. But as I mentioned, when I was shown, this crazy growing pace of paspalum, I had so many questions about it. Then James told me more about it.
James Schnable: It’s an extremely stress-tolerant grass. I mean, it’s called seashore paspalum ’cause you’ll find it right on beaches growing in the sand, with the salt.
Guangchao Sun: You can even water paspalum with sea water. That really kind of blows my mind, I really want to know more about it.
Menaka: So it grows and grows and grows, and it tolerates salt and heat. And it’s got even more going for it. Here’s James Schnable again.
James Schnable: You’re growing on sand, there really are not a lot of nutrients there. It’s essentially just, you know, tiny pieces of rock.
Menaka: In the greenhouse, that means paspalum doesn’t need a bunch of fertilizer to grow. It’s even hardy against stresses that are a bit more surprising for a beach grass.
James Schnable: Paspalum turned out to be very tolerant of cold environments, which you would not expect. It’s also tolerant of low light. There is one study looking at its ability to grow in areas that have been contaminated with crude oil. It does reasonably well with that.
Menaka: A lot to feel good about! So, Guangchao was listening — this grass seemed special. But there was something else he learned that got him even more interested.
Guangchao Sun: When I was told that paspalum is actually also a wild relative of maize, and sorghum, you know, maize and sorghum are very well known domestic crops. Right. I wonder what happened — what made these three species, right now, so different, especially between paspalum, and maize and sorghum.
Menaka: Guangchao is saying this pretty diplomatically — but basically, the maize and sorghum that we grow as crops aren’t quite as hardy as they’ll need to be as our climate changes. They’re the result of human selection in fields, not natural selection in the world.
Guangchao Sun: Maize and sorghum, you can say they are somehow spoiled, you know. We gave them enough of water, nutrients
Menaka: So over millennia, as we’ve farmed maize and sorghum, they’ve changed in ways that delete their earlier coping mechanisms. Why keep those tools around, if a farmer is always nearby with plenty of fertilizer and water?
Meanwhile, here was paspalum – a relative from the same common ancestor as corn and sorghum. And even in bad conditions, it could spread like a weed. So clearly, a lot was different between these plants.
Guangchao Sun: And that is such a big question. But at least I was wondering whether or not there are some, traits that are conserved from their common ancestor, but somehow, you know, got diverged during the long time of evolution.
Menaka: So paspalum could have slightly different mechanisms for photosynthesis and dealing with drought, or a lack of nutrients. But it’s also a pretty close relative to those two important crops.
Menaka: And so if you have a trait in paspalum, it could transfer really well to sorghum and maize.
Guangchao Sun: Yeah.
Menaka: If you think about these plants’ genomes as different drafts – so they all start at the rough draft of their common ancestor, then go through different revisions,
Menaka: Sorghum and maize are sort of later drafts of a plant and paspalum is like a slightly earlier draft, although it still exists at the same time.
Guangchao Sun: Yeah, yeah. You are right –
Menaka – GS Interview: But you could almost like, pull a good sentence from your first draft and put it in a later draft. Just something good that got lost.
Guangchao Sun: Yes, yes, exactly.
Menaka: So here was Guangchao, a new postdoc, forming this research question he was super curious about. What did paspalum hang onto in its genome, that maize and sorghum might have lost?
And remember – he had an assignment on another project. But now he also had a growing list of reasons to work with paspalum. First, it’s a super hardy, fascinating wild grass. Second, it’s related to important crops.
Guangchao Sun: And third is James told me, “Hey, we are also collaborating with the JGI, DOE and University of Georgia to sequence and, and assemble this genome. And we also get a very good annotation on the genome.” <snaps fingers> Bam. I said, “Okay, I wanna work on this.”
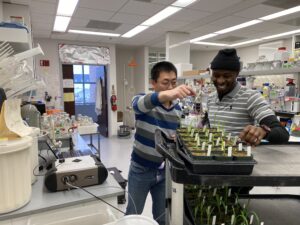
Guangchao Sun works with student Aime Valentin Nishimwe to collect data from
plants grown without enough nitrogen fertilizer. (James Schnable)
Menaka: Thank goodness, because otherwise, the second half of this episode would be quite difficult to put together. Guangchao and James found some really interesting results. Which we’ll get to in a moment. But first, a quick break.
Allison: The JGI supported this project via the Community Science Program. This program provides genomics resources for projects with Department of Energy relevance. And we accept proposals from scientists at all career stages. James Schnable, for instance, submitted the proposal for this work as a postdoc, before he’d started his own lab. So that worked out well for him – but you don’t have to take it from us. Here’s James.
James Schnable: The genome of a plant like paspalum is on the order of 700 megabases, which is 700 million A-T-C-G’s, that you have to get in the right order. Paspalum, like most plants and like humans actually carries two copies of its genome, one that it received from its mother and one that its received from its father. Paspalum in particular, because it is a self incompatible plant, we can’t generate an inbred paspalum plant, which makes assembling the genome a lot harder. It’s like trying to put together two puzzles at once that are similar but slightly different scenes and all the pieces plug in to each other. Being able to separate out and figure out which puzzle pieces go together and which ones don’t, is much harder than it would be for more grasses, and most plants, and JGI was able to do that. The big thing is just the expertise to assemble the genome itself, which I guess you could call computational tools, but it’s not really tools, it’s people who know what they’re doing. I do a lot of genomics in my lab, but genome assembly is it’s own dark art still, and JGI is really, really good at it.
Allison: You can find out more about submitting proposals to the JGI on our website. Head to joint geno-dot-me slash proposals. We’ve also got a link to our website waiting for you, wherever you’re listening to this episode. It’ll be in the episode description, or the show notes.
Menaka: This is Genome Insider, from the JGI. To recap where we’ve been so far, James Schnable and Guangchao Sun have introduced us to their very tough turf grass, seashore paspalum.
Besides being hardy, it’s related to some major crops, like corn. So James and Guangchao were looking for traits that could transfer well — from paspalum to other plants.
And they had a project set up with the JGI to look into those traits. They’d sequence the grass’ genome in full, and watch how it shifted its responses when it faced different stresses. For that, they’d use RNA sequencing, and metabolomics.
The next step was exposing paspalum to different kinds of stress: high salt, cold temperatures, less water, and less nutrients — specifically, low nitrogen and low phosphorus. Guangchao also grew maize and sorghum in these conditions to compare. He wanted to see what changed across these plants.
As they expected, the paspalum plants handled stress better. So they dove into the omics analysis to start sorting out why. That’s a dive into very deep water — into hundreds of millions of base pairs, and several different stress conditions. These are data that probably have many traits that paspalum could transfer to other grasses.
But to start, they looked closer at one idea that bobbed to the surface. <music out>
When they gave paspalum plants low water, or low nutrients, those plants survived. And again and again, those plants seemed to be stockpiling a certain molecule. It was a sugar, called trehalose. Guangchao Sun, again:
Guangchao Sun: So when we looked at the metabolomics analysis, we found that this trehalose accumulation in paspalum is about twofold higher, under nitrogen starvation or phosphorus starvation condition, compared to when it’s growing under full condition.
Menaka: Makes you wonder — was this trehalose helping these plants survive? Also, um, what is trehalose?
In terms of its chemical structure, trehalose is two glucose molecules stuck together. And it’s very good at hanging onto water. It actually shows up in the ingredients lists for some eye drops meant to treat dry eye. And Guangchao also read up on trehalose in plants.
Guangchao Sun: It’s a signaling molecule instead of a, you know a sugar molecule that provides energy in plants. And usually trehalose is involved in stress responses in plants.
Menaka: So these paspalum plants were doing something that other researchers had seen before. As the plants handled stresses, like low water, or low nutrients, they built up trehalose in a way that helped them cope. And when Guangchao looked at the genome sequence from the JGI, it seemed like this had been happening for a long time in the plants’ history.
Guangchao Sun: We found that the genes involved in trehalose metabolism are under higher selection pressure in paspalum.
Menaka: Over its evolution in wild fields, paspalum had prioritized the genes it needed, to make and use this sugar.
So, in a tidy, simplified equation, this looked like: paspalum, plus stress, equaled trehalose.
But to make this a solid finding, this team wanted to check that equation in multiple directions. Could they boost paspalum’s trehalose stockpile, and see an even better stress response? Or, in the opposite direction, could they take away that stockpile, and watch paspalum wilt in the face of stress?
Great questions. But it turns out, those checks would be really tricky to do in paspalum. Boosting or busting its trehalose stockpile would require genomic tweaks. And paspalum is a wild plant – not very tweakable. James Schnable, again.
James Schnable: One of the key challenges, and this is part of why the paper was in review for over a year, is that we couldn’t do some of the validation experiments that would be conventionally done in a study like this.
Menaka: In domesticated plants, researchers often have worked out how to transform a plants’ genome. So it’s possible to do all kinds of studies for validation. They transform a plant into a mutant, one that’s better or worse at the trait they’re testing, then see if that mutation actually matters.
James Schnable: You know, it’s sort of a gold standard for, for testing mechanisms in plants. And we couldn’t do that in paspalum. We didn’t have the capacity to generate mutants, to test what we were seeing.
Menaka: Making a paspalum mutant would be another multi-year project. So tweaking its trehalose levels to test this theory was off the table. They had to get a bit more creative. And this was just about when another researcher joined the project.
Vladimir Torres: My name is, Vladimir Torres, and I am right now working as a postdoc, in James Schnable’s Lab here in the University of Nebraska Lincoln.
Menaka: Vladimir did lots of validation experiments. But he didn’t transform paspalum.
James Schnable: Instead, what we were able to do is, create the same mechanism in corn and then show with mutants in corn that, aha, this works in corn.
Menaka: So there was a big benefit there – other researchers have already worked out how to transform corn, so Vladimir could work with mutants to boost or bust their trehalose stockpiles, and see what happened.
Vladimir Torres: Plus, it’s always more fun and more interesting to see how is that in some crops, in this case corn, but could be sorghum, or next time, rice.
Menaka: Yeah — it’s also neat that these validation studies were along the lines of the whole goal of this project — finding traits from wild plants that could also help domesticated crops. And Vladimir’s help made a big difference in finishing these studies.
Guangchao Sun: Those experiments are not easy to do, even though it looks pretty straightforward. It takes a lot of practice and lots of failures to really get to that stage. But he really made it, he’s very good.
Menaka: When I talked with Vladimir, he talked me through some photos of the corn plants he worked with.
Vladimir Torres: Those are really good photographs. Because I love the plants. And basically we have really good facilities, like greenhouse facilities here in Nebraska, and the greenhouses are just amazing. The people who are working there is, is awesome. And they take care of these plants really well. So, about this photo…
Menaka: Each corn plant is growing in its own little white bucket, in an indoor greenhouse. The corn plants look quite happy in their little indoor cornfield. In one photo, Vladimir is actually standing on a step stool to reach the top of a plant.
Menaka: And how tall are these?
Vladimir Torres: Wow. These were, I don’t know, probably more than three meters.
Menaka: That seems really tall <laugh>
Vladimir Torres: That’s really tall.
Menaka: And there’s something else interesting about some of the plants we’re looking at.
Vladimir Torres: So basically all these plants are carrying the mutation that we are interested in. We initially aimed to grow 300 plants. And then from them, we select, or we genotype, a few of them to confirm, that some of them had a mutation. So basically when we got some, 24 plants that got a mutation, we, uh, move forward only with those plants.
Menaka: Ok these are the 24 chosen, huh?
Vladimir: Yes <laughs>.
Menaka: So the plants they moved forward with, <music up> were mutated. We’ll explain that mutation soon. For now, what’s important is that these plants were changed in a way that would help this team ask a question — about that trehalose stockpile that seemed to help paspalum plants. Could that stockpile also help corn grow, with less water, or less nutrients?
To answer that question, this team designed experiments that would essentially give them switches to control the effects of stockpiling trehalose. They had an on switch, as well as an off switch.
Let’s start with setting up that on-switch. So this takes us away from the mutant plants for a minute. At first, Guangchao actually tried the simplest idea he could think of to boost that trehalose stockpile.
Guangchao Sun: So I applied trehalose to the soil directly, and it turned out the plants are stressed out because trehalose is also causing a big osmotic stress in the soil. So maize and sorghum, they cannot deal with that. They cannot even take water from the soil. So , they dried out pretty fast.
Menaka: It wasn’t possible to just give plants more of this sugar in the soil. And it was kind of complicated to try to get these plants to make more trehalose.
Guangchao Sun: So I thought about it, about it in the opposite way. What if I could, you know, stop the degradation of trehalose.
Menaka: And there, his idea was basically, to get a plant to save up this sugar, he could help it spend less. And plants spend, or break down trehalose with a specific enzyme. It’s called trehalase. So Guangchao looked into ways of affecting trehalase.
Guangchao Sun: I just searched online, see if there’s any specific inhibitors of trehalase. And then I found validamycin A.
Menaka: Validamycin A is an antibiotic that stops the enzyme trehalase from breaking down the sugar trehalose. It forces plants to save their trehalose. And when Guangchao applied that antibiotic to plants,
Guangchao Sun: I saw that maize and sorghum is, you know, growing better than before, is healthier, greener, and it was accumulating more biomass than before, And I was, I got, you know, so excited about it.
Menaka: So that took care of the on-switch for the trehalose stockpile. <click>
Next, the off-switch. To get an off-switch they could toggle, this team had to wire up a few more connections to this trehalose stockpile. Based on the literature, they figured out that saving up trehalose boosted an important process where plants reuse bits and pieces of their structures. Guangchao told me you can actually see signs of this for yourself.
Guangchao Sun: If you have grown plants before, you see that, you know, usually the first leaf will wilt, and it’ll dry out, when the second leaf starts emerging.
Menaka: So as that first leaf is starting to die off, the plant is also starting up a process to re-use nutrients, and funnel them from old leaves to new ones. That process is called autophagy. The name autophagy is from the Ancient Greek for self-devouring. This is a process all kinds of cells do, in both plants and other organisms.
Guangchao Sun: So autophagy is something that, you know, basically recycles those nutrients in the first leaf and, and, and sends it back to the second and third, you know, to support the growth of the plants.
Menaka: So it’s like recycling at a cellular level, but then it helps the plant grow, eventually
Guangchao Sun: That’s right. That’s right. So it recycles these parts and, and then breaks them down.
Menaka: And the idea was that storing up trehalose boosted this autophagy process. To dim that effect, with an off-switch, they went after autophagy itself. And that’s where the mutant corn plants come in. So these are the mutant corn plants that Vladimir worked with. They’ve got a specific mutation in a single gene that’s called ATG-12.
Guangchao Sun: When we employ this ATG-12 mutant in maize, right, so these mutants are autophagy deficient, basically.
Menaka: Those ATG-12 corn plants don’t perform autophagy as well — so for them, the effects of a trehalose stockpile are switched off.
And with that, the switches toggled both ways. So with an antibiotic as an on switch, and a genetic mutation as an off switch, Vladimir was ready to test whether or not the trehalose stockpile mattered for corn. He did stress experiments, the same way that Guangchao started out in paspalum.
He said when he was doing these experiments, he could kind of see what was going on, even before the analysis was all done. Corn plants sort of wear their feelings on their leaves.
Vladimir Torres: So basically when you are applying low nitrogen to corn, you can see this yellow color in the leaves that is so dramatic. And also the biomass, or the growth stops because, just because nitrogen is so important for the plant.
Menaka: So corn without nitrogen looks really bad. But in experiments, they flipped the on-switch for the trehalose accumulation – using that antibiotic. And even in low-nitrogen conditions, the corn looked much better.
Vladimir Torres: Because basically, when you apply validamycin A, you are accumulating trehalose and then you are triggering the autophagy, and then you are also accumulating more, biomass. So you can see that.
Menaka: But with the mutant – where the off-switch is always flipped off – adding the antibiotic didn’t have the same effect.
Vladimir Torres: So that’s why we conclude that this increase in the biomass in low nitrogen was, due to autophagy activity.
Menaka: Got it. Because like, if you can turn it off, then you understand how you could turn it on. That makes sense to me.
Vladimir Torres: Yeah, exactly.
Menaka: The theory they went in with did stand up in these experiments.
James Schnable: It looks like what’s happening is, this trehalose is causing corn to up-regulate its sort of natural recycling program so it can reuse existing nitrogen within the cell more efficiently.
Menaka: They definitely learned something that could be useful in the future.
James Schnable: I would say that we learned from a wild plant, a new way to turn on a recycling mechanism in corn so that corn can use less fertilizer and still do all the things it needs to do to be a successful corn plant.
Menaka: And how cool – that the idea came from an extremely successful wild grass — one that’s managed to survive on salty, sandy beaches, as well as those World Cup fields of Qatar.
So at the end of my conversation with Guangchao, I had to ask him one last very important question, about that wild grass that this whole story started with.
Menaka: My other question was if your kids watched the World Cup and if you pointed out the grass to them,
Guangchao Sun: So, I really wanted to. They are so little now, but I did tell them, I did tell them, do you see the green stuff? I sequenced it. I can see confusion on their face, but, you know, somehow when they grow up, I will let them know, you know. <laughs>
Menaka: And who knows – hopefully, sometime in Guangchao’s kids’ lifetimes, we’ll have more crops with the traits that made paspalum a great choice for the World Cup. The way James said it, More crops is good. But the water and fertilizer they need also matter — so growing tougher crops is key for our future.
James Schnable: More crops with less impact, even better.
Menaka: Productive crops that are easier on the planet, a real win win. Or, in soccer speak — Goooaaaal!
<THEME>
Menaka: So again, that was James Schnable and Vladimir Torres from the University of Nebraska at Lincoln. After his postdoc, Guangchao Sun joined the Mayo Clinic as a bioinformatician.
They published this work in the journal Nature Communications. We’ve linked to that paper in the show notes.
The JGI enabled this work via the Community Science Program. You can find out more about this work, and the Community Science Program, at the JGI website – there are links in the show notes, as well as a transcript online.
This episode was written, produced and hosted by me, Menaka Wilhelm. I had production help from Allison Joy, Massie Ballon, Ingrid Ockert and Graham Rutherford.
We had music in the middle of this episode by Cliff Bueno de Mesquita, who’s a multitalented postdoc at the JGI.
If you liked this episode, help someone else find it! Tell them about it, email them a link, or leave us a review wherever you’re listening to the show.
Genome Insider is a production of the Joint Genome Institute, a user facility of the US Department of Energy Office of Science located at Lawrence Berkeley National Lab in Berkeley, California.
Thanks for tuning in – until next time!
Show Notes
- Publication: Sun, G., Wase, N., Shu, S. et al. Genome of Paspalum vaginatum and the role of trehalose mediated autophagy in increasing maize biomass. Nat Commun 13, 7731 (2022). doi: 10.1038/s41467-022-35507-8
- Submit your own proposal to work with the JGI!
- Join us at the JGI User Meeting this August
- Episode Transcript
- Paper: Genome of Paspalum vaginatum and the role of trehalose mediated autophagy in increasing maize biomass
- Phytozome: Paspalum vaginatum data
- Our contact info:
- Twitter: @JGI
- Email: jgi-comms at lbl dot gov